Stratigraphy & Biostratigraphy Explainer: Reading Rocks Through Their Fossils
- jordanhealey5
- Sep 26, 2022
- 8 min read
What is Stratigraphy?
Stratigraphy is the science of correlating, ageing and characterising rock units, using the information locked into place when they form. As sedimentary deposits accumulate and slowly transform into rocks, they do so in layers often compared to the pages in a book. Every layer tells a story about the timing and location during deposition, and the further down you go, the further back in time you are looking. Nicolas Steno, the father of stratigraphy, first outlined its four guiding principles back in 1669. These are still used to understand rock strata (layers) in the field and to interpret geological maps.
Steno’s four principles state that:
1.) in undeformed strata, the youngest unit lies at the top and layers get progressively older further down
2.) sedimentary layers are originally deposited horizontally
3.) deposits extend continuously within the confines of where they are deposited i.e. the shape of a basin
4.) if a feature or deposit is cross-cut by another, it must have formed earlier.
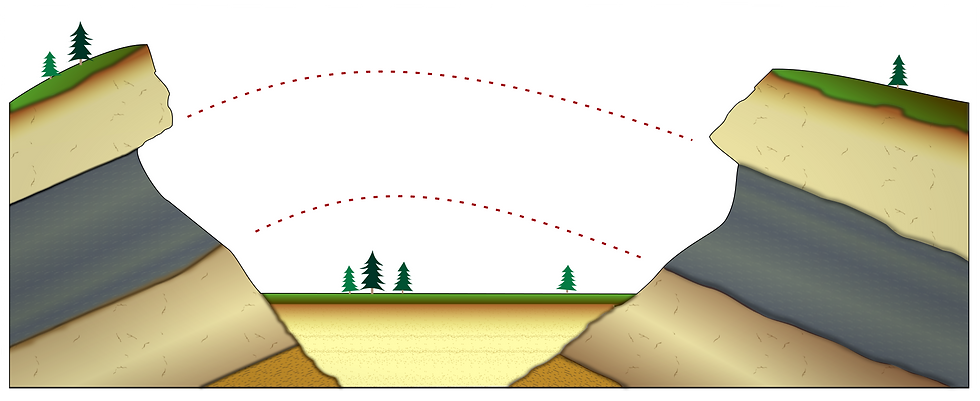
Illustration of the third principle of stratigraphy - lateral continuity. The “missing” rock has been eroded but is inferred to have been connected in the past.
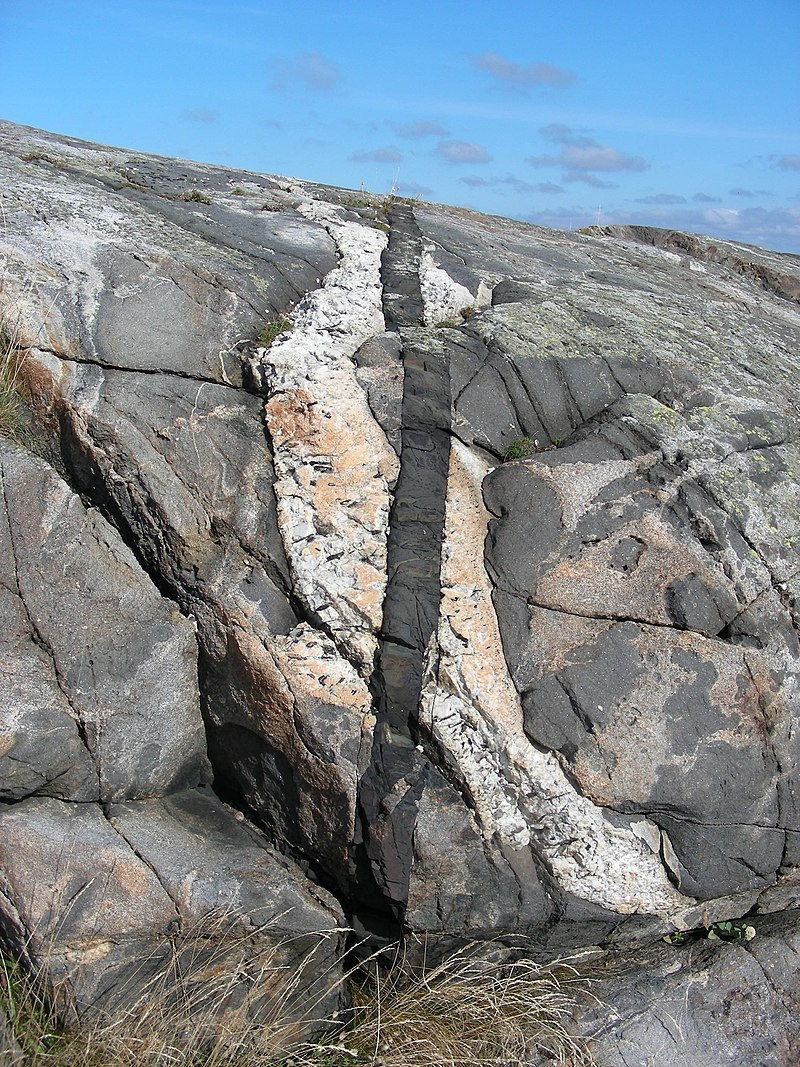
Rock section highlighting cross-cutting relations, the fourth principle - light grey rock is cross-cut by a younger white intrusion. Both features are cross-cut by a thin black intrusion, which must be the youngest overall. This basic field observation starts to portray a sense of the evolution of these rocks. Two pulses of magma were injected into the crust and travelled to the surface exploiting fractures/structural weaknesses.
Geologic and Earth surface processes often complicate relations as rocks are subject to immense pressure and temperature conditions during burial, uplift, folding, faulting, weathering, and erosion over enormous timespans. Rocks rarely exist in their original, unaltered state, especially older ones. Geologists rely on a series of indicators to correlate between rocks and determine their evolution on small and local or much larger regional scales, which is where stratigraphy proves to be so valuable.
James Hutton was the first to describe rocks as the products of millions of years of gradual change. He came to this realisation after observing a stratigraphic relation called an angular unconformity, where a rock bed overlies another in a different orientation. The site where Hutton first noticed this, Siccar Point in Scotland, is now among the most famous rock outcrops in the world due to its historical significance. Unconformities are gaps in the geologic record caused by erosion or a hiatus in the deposition.
There are two main ways to age rocks and achieve the primary aim of stratigraphy: absolute ages provide a number e.g. 20 million years +/- 2 million years (often relying on geochemical techniques), and relative ages give broader estimations or ranges e.g. in the image above, where the cross-cutting provides a rough outline for the order of events without specific age values.
It is important to weigh up the pros and cons of which type would be needed for a project as absolute dating is usually time-consuming and expensive, and not all rocks meet the conditions required for absolute ageing. Sometimes being in the field and mapping is sufficient to understand the geologic history of a location and the relative timing of events. Of course, it’s common to combine the two to give the broadest picture possible. Different stratigraphic techniques are often used together to constrain the positions of rocks in time and space as much as possible.
The Geological Time Scale
Correlating rocks enables researchers to piece together the Earth’s past through its rock record and divide its 4.6 billion-year history. Stratigraphy pieces together our planet's story from the incomplete record. The geological time scale is the culmination of all this work and is to geologists what the periodic table is to chemists.
The time scale divides history into different subdivisions of time, defining subdivisions with key, global markers such as the first or last appearance of a fossil or the flipping of the Earth’s magnetic field (which causes magnetic minerals to change their alignment). Geologic time comprises eons, eras, periods, and series going from the longest to shortest subdivisions. The nested subdivisions that form the basis of the scale are similar to how biologists classify life into a taxonomic rank, with the most significant events like mass extinctions delineating a higher-ranked boundary.
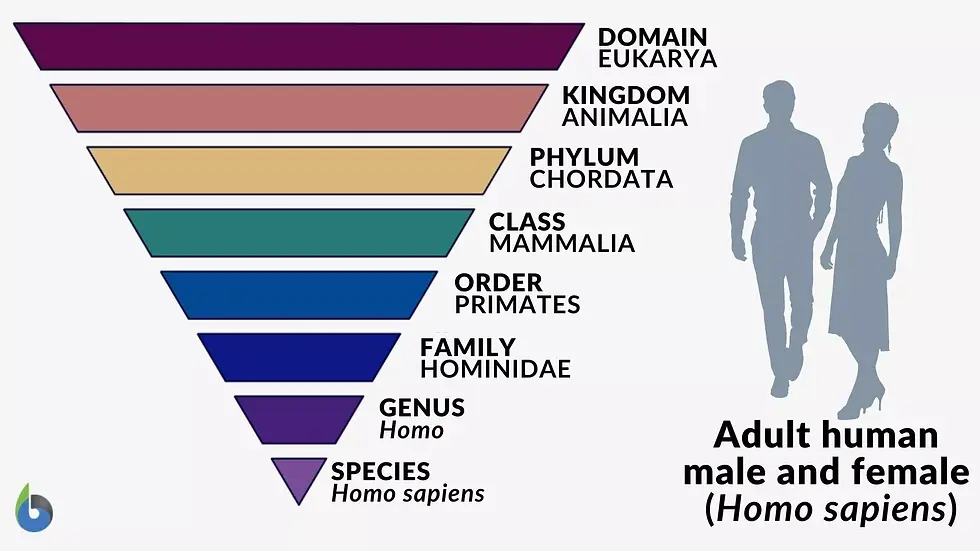
Living animals, plants, and fossils are subdivided according to key characteristics selected by biologists to identify them. Each rank down refers to a smaller group, similar to how geoscientists classify the timescale.
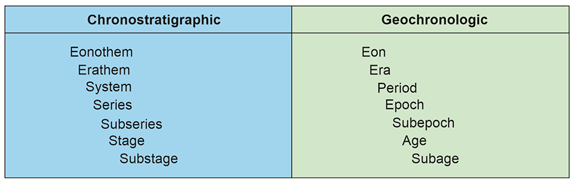
Hierarchy of stratigraphic classification. “Chronostratigraphic” column indicates the terms used to define rock boundaries that mark changes in time, aged using various techniques. The “geochronologic” column refers to the time itself.
Chronostratigraphy is the branch of stratigraphy that divides up Earth's history, using various stratigraphic techniques to correlate rocks in different locations and understand the conditions during their formation. Stratigraphers divide up the timescale according to major events such as extinctions, environmental changes, and other Earth System changes, which leave behind a distinct chemical or fossil-based fingerprint in their wake.
The most famous example of a boundary is the mass extinction caused by the meteorite impact 66 million years ago, at the end of the Cretaceous Period in the Yucatan Peninsula, Mexico. The impact ejected enormous amounts of material from the meteor and the Earth’s crust into the air and dispersed it in a layer, getting thinner with greater distance from the impact site. This event is associated with a characteristic signature - an iridium layer formed as the material rained back down onto the surface and became part of the sedimentary record. This layer meets the requirements to be a primary marker, according to the International Commission on Stratigraphy, the organisation of scientists responsible for maintaining the timescale. Secondary markers include fossil evidence that records the mass extinction, including the disappearance of dinosaurs on land and ammonites in the oceans.
What is biostratigraphy?
As the name gives away, biostratigraphy involves using past life (fossils) to age and correlate the rocks around us. It has been around since the 19th century, before the age of the Earth was known, and has become the most widely used way of organising the geologic time scale since the Cambrian, where the reliable fossil record began 540 million years ago. While more limited than, for example, radioisotope dating (which can give a specific age within some margin of error), fossils are widespread and easier to disentangle.
There are several ways that fossils can act as stratigraphic markers, but the most common is their first or last appearance in a layer. A first occurrence (first appearance datum or FAD) indicates a newly evolved species or an environmental/migration-related change resulting in the species becoming part of the sedimentary record. The last appearance datum (LAD) may indicate an extinction or local reorganisation of migration patterns that lead to a species moving away from a geographic region. Matching the first or last appearance of fossil markers across rocks in different parts of the world enables them to be correlated as they are assumed to have evolved at the same geologic time.
Fossils provide the best way of identifying the ages of rocks and correlating between them due to their widespread occurrence in sedimentary rocks since the Cambrian explosion. For example, if a sedimentary rock unit contains ammonite fossils, it must have been deposited when ammonites were living, between ~400 million years ago and 66 million years ago. By identifying an exact species or genus, the age can be further constrained - ammonites are an especially useful fossil for identifying rocks that are Jurassic or late-Triassic in age and putting rocks deposited at these times into tighter subdivisions. A species that marks a new era, period, or series in time is referred to as an index fossil.
Other excellent timekeeper fossils include conodonts; the most widely used markers between ~500-200 million years ago, graptolites; trilobites; and foraminifera, whose shells also preserve valuable isotope data that tells us about the climate when it was alive. These fossils contain some combination of these characteristics, making them valuable to geologists. While there is no perfect fossil for correlation, these are the most effective ones that meet the criteria scientists have developed over the centuries.
For an organism to be an index fossil, it needs several key characteristics. Most obviously, the fossil must have a high preservation rate and hard parts that can be identified even millions of years after the organism dies. It could meet all the other requirements but would be no good for correlation if it isn’t abundant or a whole skeleton is needed to identify in a meaningful way. It should also be geographically widespread and, ideally, preserved in different environments. Typically, ocean life is far better at meeting these criteria, which explains why most index fossils used for the geologic time scale are marine species.
As mentioned previously, fossils are useful because their first or final appearance gives a good indication of when the rock itself formed. If it was rapidly evolving, new species mark intervals in time more precisely and with better resolution.
Furthermore, a species should have preserved features that lock in evolutionary changes. Ammonites are such famous timekeepers for this reason. The size and patterns of ammonite fossils, which also happen to be exceptionally well-preserved, can be used to recognize different species, so simple field descriptions unravel lots of information.
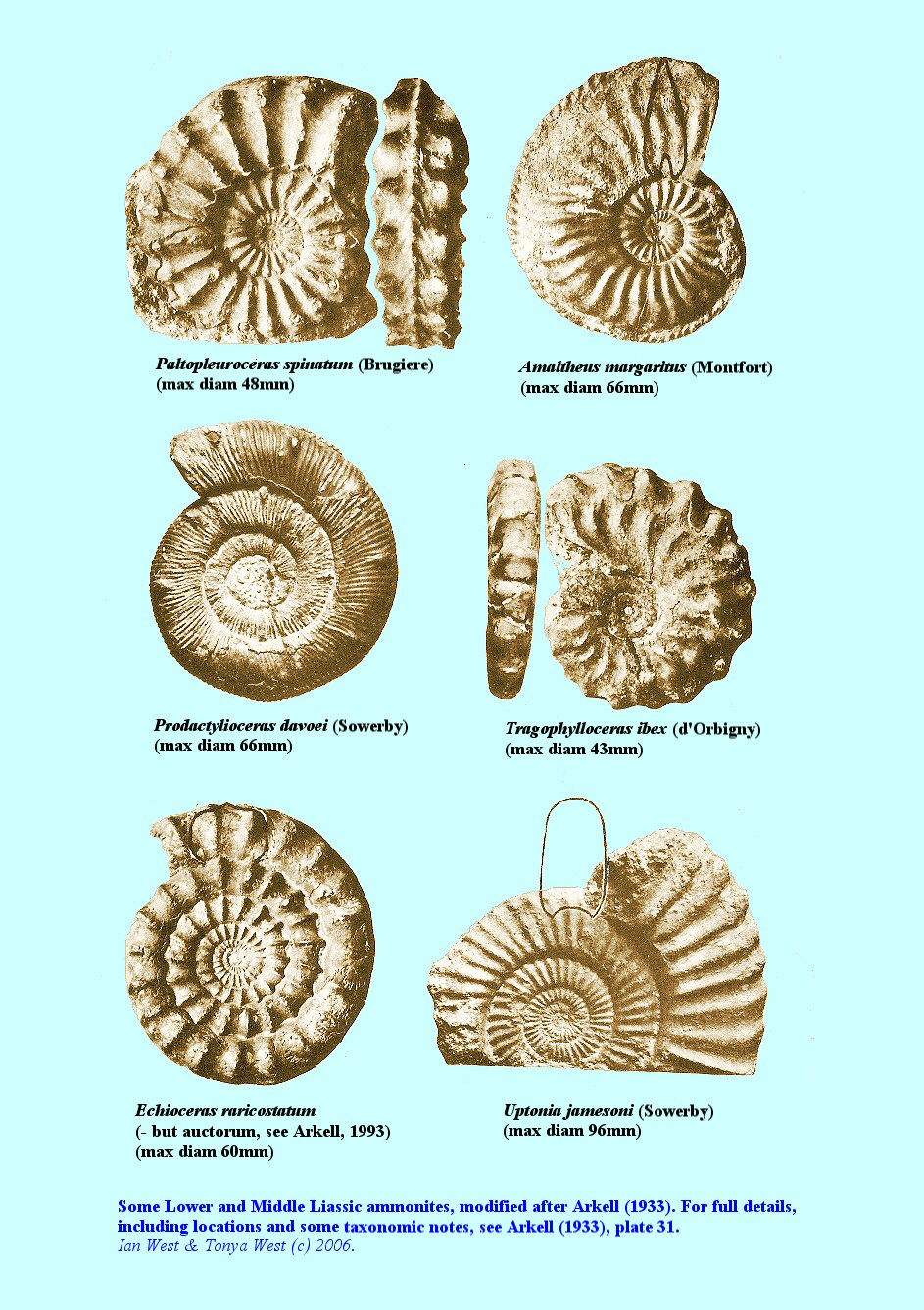
Some common species of ammonite. Major characteristics in their shells can be used to distinguish between them and assign relative ages.
Fossils and Continental Drift
The science of stratigraphy involves piecing together the geological record across the world. When Alfred Wegener first proposed his theory of continental drift in the early 20th century, he sought to explain why the continents seen on a map fit together like a jigsaw. This pattern led him to consider the possibility that they have moved apart over long timescales. Beyond this possibly coincidental pattern, he discovered more and more pieces of evidence to bolster his initially unpopular hypothesis.
One of the first pieces of evidence was the occurrence of fossils that matched across the continents, implying that, when they were alive, they could freely move between the continents. Researchers were not convinced at the time, believing that sea-level changes, which would periodically submerge and expose land, explained these observations.
Proof of continental drift eventually came in the 1960s, long after Wegner's death. Magnetostratigraphy was used to prove seafloor spreading after decades of skepticism. The gradual movement of tectonic plates over long timescales is one of the main reasons life came to be on our planet. The earth system is constantly kept in check by the movement of these plates, allowing life to evolve towards the complexity we see today.
The theory proposed by Wegner has also found enormous value in reconstructing the movement of tectonic plates over time (a discipline called palaeobiogeography). For example, building on his observations enabled scientists to reconstruct Pangea and document its assemblage and break up.
There are many different types of stratigraphy. These include bio-, magneto-, litho-, chrono-, Astro-, and sequence stratigraphy - all used to correlate and date rocks. Of all these techniques, biostratigraphy is the most widely used to mark and characterize major geologic events over the past 540 million years. The fossils lock in so much information about the environment at the time of deposition, clues about global climate and the carbon cycle, extinctions, and far more about deep time.
Biostratigraphy is an extraordinary technique that enables scientists to unpack so much information about our planet, piecing the remains of life from millions of years ago into a larger image of the world during these times. It offers answers to questions like what environment a rock was deposited in, when it was deposited, how two rock units separated by an ocean may be linked, and what the conditions were like at the time a fossil or group of fossils lived.
This article only scratches the surface of the fascinating topic, and how powerful it is, especially when combined with other similar techniques. I plan to write more about other stratigraphic tools and begin to see how their relationship unfolds to inform us about the Earth and its equally fascinating story.
___________________________________________________________________________
Useful video explainer on biostratigraphy https://www.youtube.com/watch?v=wNT_JrKCeJI
Comments